Exploting sound waves to improve optical communication
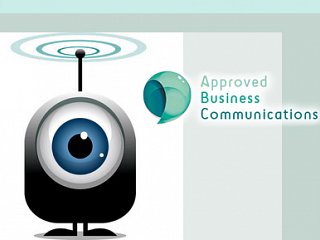
A new study shows that sound waves can be exploited to create ultraminiature optical diodes small enough to be placed onto a computer chip. Such diodes, also defined optical isolators, may contribute to solving the issue if data capacity and system size challenges for photonic integrated circuits, which represent the light-based equivalent of electronic circuits used for communications and computing
Isolators are one-way tools that resemble electronic diodes. Their main role is to protect laser sources from back reflections and play a key role in routing light signals around optical networks. Nowadays, the main technologies that specialise in the creation of such one way ‘nonreciprocal’ tools need materials that change their optical properties in response to magnetic fields, the researchers explained.
“There are several problems with using magnetically responsive materials to achieve the one-way flow of light in a photonic chip,” explained the co-author of the study Gaurav Bahl , mechanical science and engineering professor at Illinois. “First, industry simply does not have good capability to place compact magnets on a chip. But more importantly, the necessary materials are not yet available in photonics foundries. That is why industry desperately needs a better approach that uses only conventional materials and avoids magnetic fields altogether.”
The authors explain how they exploit the minuscule coupling between light and sound to provide a unique solution that enables one-way devices to work with nearly any photonic material. The study was published in the journal Nature Photonics
However, the physical size of the device and the availability of materials are not the only problems in the specific market, the researchers said.
“Laboratory attempts at producing compact magnetic optical isolators have always been plagued by large optical loss,” explained graduate student and lead author Benjamin Sohn. “The photonics industry cannot afford this material-related loss and also needs a solution that provides enough bandwidth to be comparable to the traditional magnetic technique. Until now, there has been no magnetless approach that is competitive.”
The new device is only 200 by 100 microns in size - which is about 10,000 times smaller than a centimeter squared – and made of aluminum nitride, a transparent material that is compatible with photonics foundries and transmits light.
“Sound waves are produced in a way similar to a piezoelectric speaker, using tiny electrodes written directly onto the aluminum nitride with an electron beam. It is these sound waves that compel light within the device to travel only in one direction. This is the first time that a magnetless isolator has surpassed gigahertz bandwidth,” Sohn said.
The researchers are now seeking means to increase bandwidth or data capacity of these isolators and believe that they will succeed in their attempt. Once perfected, they predict transformative applications in photonic communication systems, gyroscopes, GPS systems, atomic timekeeping and data centers.
“Data centers handle enormous amounts of internet data traffic and consume large amounts of power for networking and for keeping the servers cool,” Bahl said. “Light-based communication is desirable because it produces much less heat, meaning that much less energy can be spent on server cooling while transmitting a lot more data per second.”
Aside from the technological potential, the researchers stress the fascinating side of the fundamental science behind this advancement.
“In everyday life, we don’t see the interactions of light with sound,” Bahl said. “Light can pass through a transparent pane of glass without doing anything strange. Our field of research has found that light and sound do, in fact, interact in a very subtle way. If you apply the right engineering principles, you can shake a transparent material in just the right way to enhance these effects and solve this major scientific challenge. It seems almost magical.”
The research was supported by the United States Defense Advanced Research Projects Agency and the Air Force Research.
Written by: Pietro Paolo Frigenti